Shvo catalyst
![]() | |
Names | |
---|---|
IUPAC name
1-Hydroxytetraphenylcyclopentadienyl-(tetraphenyl-2,4-cyclopentadien-1-one)-μ-hydrotetracarbonyldiruthenium(II) | |
Identifiers | |
104439-77-2 ![]() | |
Properties | |
C62H42O6Ru2 | |
Molar mass | 1085.13 |
Appearance | orange solid |
Melting point | 223 to 227 °C (433 to 441 °F; 496 to 500 K) |
polar organic solvents | |
Except where otherwise noted, data are given for materials in their standard state (at 25 °C [77 °F], 100 kPa). | |
![]() ![]() ![]() | |
Infobox references | |
The Shvo catalyst, named after Youval Shvo, is an organoruthenium compound that is used for transfer hydrogenation. Related derivatives are known where p-tolyl replaces some of the phenyl groups. The compound is of academic interest as an early example of a catalyst for transfer hydrogenation that operates by an "outer sphere mechanism." [1]
Commonly, industrial hydrogenation processes involve heterogeneous catalysts containing one or more transition metals.[2] While this approach facilitates efficient production on an industrial scale, heterogeneous catalysis is not always suitable for the asymmetric synthesis of more structurally complex molecules, which demands the increased versatility precluded by necessary interaction with a solid metal surface.
One half of the 2001 Nobel Prize in Chemistry was awarded jointly to William S. Knowles and Ryoji Noyori "for their work on chirally catalysed hydrogenation reactions", and the other half was awarded to K. Barry Sharpless "for his work on chirally catalysed oxidation reactions", highlighting the growing importance of homogeneous catalysis as an industrially applicable redox technique for asymmetric synthesis.[3] Shvo's catalyst represents a subset of homogeneous hydrogenation catalysts that involves both metal and ligand in its mechanism, and it therefore presents unique opportunities for customizing selectivity.
Synthesis, structure, reactivity
The complex was originally prepared by the reaction of diphenylacetylene and triruthenium dodecacarbonyl. This synthetic route is efficient, despite the complicated pathway, which includes formation of cyclopentadienone-like ligands.[4] Related syntheses use the preformed cyclopentadienone.[5] A related iron analogue is also known, see Knölker complex.
The compound contains a pair of equivalent Ru centres that are bridged by a strong hydrogen bond and a bridging hydride. In solution, the complex dissociates unsymmetrically:
- (η5-C5Ph4O)2HRu2H(CO)4 → (η5-C5Ph4OH)RuH(CO)2 + (η6-C5Ph4O)Ru(CO)2
The hydride (η5-C5Ph4OH)RuH(CO)2 transfers H2, especially to polar substrates such as ketones and iminium cations. The cyclopentadienone species (η6-C5Ph4O)Ru(CO)2 abstracts H2 from substrates.[6]

Discovery
In the early 1980s, while studying transfer dehydrogenation reactions catalyzed by triruthenium dodecacarbonyl, it was noted by Youval Shvo that both rate of reaction and catalytic turnover were drastically improved through the use of diphenylacetylene as hydrogen acceptor.[7] A series of judicious experiments demonstrated that under the reaction conditions, diphenylacetylene was reacting with the complex to form cyclopentadienone ligands in situ. The complexes with cyclopentadienone were shown to be the source of the improved outcomes.[8]
In 1986, Shvo and others reported that they had characterized this new class of ruthenium complexes which were catalytically active in the hydrogenation and dehydrogenation of numerous functional groups.[4]
Synthesis

Shvo's catalyst is typically synthesized using tetracyclone and triruthenium dodecacarbonyl, which are refluxed together in a dry aromatic solvent such as toluene for at least 2 days. After forming the monomeric species, the bridged hydrogen dimer is formed by refluxing in the presence of a hydrogen donor, such as isopropanol.[1]
Reactions
Structural basis of reactivity

When in solution, Shvo's catalyst dissociates into two unequal halves: one contains both hydrogen atoms from the dimer, and the other becomes coordinatively unsaturated. The hydrogen-containing complex performs hydrogenation through concerted addition of both H+ and H- to the double bond, while the unsaturated complex abstracts hydrogen from a suitable donor or from H2 gas itself. Together, the components work in tandem to efficiently transfer H2 from one molecule to another, bringing about the redox reaction.
Applications
Hydrogenation
In the presence of a suitable hydrogen donor or hydrogen gas, Shvo's catalyst is useful for the reduction of several functional groups. Recently this catalyst was demonstrated to be effective at hydrogenating bio-oil in order to improve its quality as a fuel and promoting the hydrolysis of complex carbohydrates into monomeric sugars.[9]
Hydrogenation of carbonyls
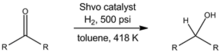
While the catalyst was initially studied in the context of reduction of aldehydes and ketones, further examination in the area has revealed ways to tailor reactivity of the complex to achieve desired outcomes. The most notable example is the use of chiral ligands to achieve enantioselective reduction with ratios up to 99:1.[10]
Hydrogenation of alkenes

Catalytic reductions of alkenes with different degrees of substitution have been reported, many of which give quantitative yields.[1]
One obstacle to the use of Shvo's catalyst in the hydrogenation of alkynes is its propensity to bind the alkyne quite tightly, forming a stable complex that gradually poisons the catalyst.[11]
Hydrogenation of imines
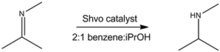
A comparative study analyzing the rate of hydrogenation among substrates determined that imines reacted more rapidly than their corresponding aldehyde counterparts by a factor of 26.[12]
Dehydrogenation
When a hydrogen donor other than H2 gas is used for a hydrogenation reaction, it becomes dehydrogenated by the catalyst as a result. The catalytic process can thus be seen as a dehydrogenation if the hydrogen donor is the target of the method, using an auxiliary hydrogen acceptor instead. For example, "Isopropanol is used with Shvo's catalyst to reduce 2-butene to butane," and "2-butene is used with Shvo's catalyst to oxidize isopropanol to acetone," are describing the same process.
It is worth noting that a suitable hydrogen acceptor/donor for a given reaction must be chosen to provide sufficient thermodynamic incentive for the desired hydrogen transfer. It is possible to transfer hydrogen in an intramolecular fashion, for example when converting allylic alcohols to ketones.[13]
Additions
When used with certain reactants, the catalyst facilitates an addition reaction through oxidation and/or traps the product through reduction; in other words, the hydrogen donor and acceptor can be the same molecule at separate points on the reaction timeline.
Oxidative coupling of primary alcohols to esters
After primary alcohols are oxidized to aldehydes via the catalyst, the aldehydes formed undergo a Tishchenko reaction to form the corresponding ester.[14]
Amination of propargyl alcohols
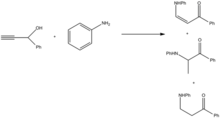
Addition of the amine is facilitated through oxidation to the ynone, followed by reduction of the product. Multiple products are possible due to amine additions at different sites; modified ligands can be used to favor desired products over others.[15]
Alkylation of amines

Another case of "hydrogen borrowing", the alkylation of amines using other amines is also promoted by Shvo's catalyst. The reaction proceeds through oxidation to an imine, which allows nucleophilic attack, followed by an elimination step and reduction of the double bond.[16]
Mechanism
Carbonyl reduction, alcohol oxidation
The exact mechanism of hydrogenation reactions performed using Shvo's catalyst has been a matter of debate, broadly between two alternative descriptions of the double bond's interaction with the complex at the rate-determining step. The proposed alternatives are an inner-sphere mechanism, where the transition state involves interaction with the metal only, and an outer-sphere mechanism, in which the cyclopentadienone oxygen also interacts in the transition-state.
Two sets of experiments support the latter explanation. Kinetic isotope studies provide evidence of a concerted transfer due to strong rate influence from both the ligand -OH and the metal hydride.[17][18] Additionally, computational evidence suggests that the transition state for an inner-sphere mechanism is disfavored over the outer-sphere mechanism by a large energy difference, which is true for carbonyl substrates[19] as well as imines, alkenes, and alkynes[20]

References
- 1 2 3 4 Brian L. Conley, Megan K. Pennington-Boggio, Emine Boz, and Travis J. Williams "Discovery, Applications, and Catalytic Mechanisms of Shvo’s Catalyst" Chemical Reviews, 2010, volume 110, pp. 2294–2312. doi:10.1021/cr9003133
- ↑ Sanfilippo, D. and Rylander, P. N. 2009. Hydrogenation and Dehydrogenation. Ullmann's Encyclopedia of Industrial Chemistry. doi:10.1002/14356007.a13_487.pub2
- ↑ "Press Release: The 2001 Nobel Prize in Chemistry". Nobelprize.org. Nobel Media AB 2013. Web. 1 Dec 2013. http://www.nobelprize.org/nobel_prizes/chemistry/laureates/2001/press.html
- 1 2 Shvo, Y.; Czarkie, D.; Rahamim, Y. (1986). "A new group of ruthenium complexes: structure and catalysis". J. Am. Chem. Soc. 108 (23): 7400–2. doi:10.1021/ja00283a041.
- ↑ Lisa Kanupp Thalén, Christine Rösch, and Jan-Erling Bäckvall (2012). "Synthesis of (R)-2-Methoxy-N-(1-Phenylethyl)Acetamide via Dynamic Kinetic Resolution". Org. Synth. 89: 255–266.
- ↑ Joseph S. M. Samec and Jan-E. Bäckvall “Hydroxytetraphenylcyclopentadienyl(tetraphenyl-2,4-cyclopentadien-1-one)hydrotetracarbonyldiruthenium(II)” Encyclopedia of Reagents for Organic Synthesis, 2008 John Wiley & Sons. doi:10.1002/047084289X.rn01063
- ↑ Y. Blum, D. Reshef, and Y. Shvo. H-transfer catalysis with Ru3(CO)12. Tet. Lett. 22(16) 1981, pp. 1541-1544.
- ↑ Blum, Y.; Shvo, Y. Isr. J. Chem. 1984, 24, 144.
- ↑ Busettoa, L., Fabbrib, D., Mazzonia, R., Salmia, M., Torrib, C., and Zanottia, V. Fuel 2011, 90(3), pp. 1197-1207.
- ↑ Noyori, R. et al. Journal of the American Chemical Society 1995 vol. 117 pp. 7562-7563.
- ↑ Shvo, Y., Goldberg, I.,Czerkie, D.,Reshef, D., and Stein, Z. Organometallics 1997 16(1) pp. 133-138
- ↑ Casey, C.P., Singer, S.W., Powell, D.R., Hayashi, R.K., and Kavana, M. Journal of the American Chemical Society 2001 vol. 123 pp. 1090-1100 doi:10.1021/ja002177z
- ↑ Bäckvall, J.-E., and Andreasson, U. Tet. Lett. 1993 vol. 34, p. 5459.
- ↑ Blum, Y.; Shvo, Y. J. Organomet. Chem. 1984, 263, 93.
- ↑ Haak, E. Eur. J. Org. Chem. 2007, 2815.
- ↑ Hollmann, D.; Bahn, S.; Tillack, A.; Beller, M. Angew. Chem. Int. Ed. 2007, 46, 8291.
- ↑ Casey, C. P., Singer, S., Powell, D. R., Hayashi, R. K., and Kavana, M. J. Am. Chem. Soc. 2001, 123, 1090.
- ↑ Johnson, J. B., and Bäckvall, J.-E. J. Org. Chem. 2003, 68, 7681.
- ↑ Comas-Vives, A., Ujaque, G., and Lledo´s, A. Organometallics 2007, 26, 4135-4144.
- ↑ Comas-Vives, A., Ujaque, G., and Lledo´s, A. Organometallics 2008, 27, 4854–4863.